Self-organization of multicellular systems by mechanical forces
A03-1
Autonomous maintenance of tissue robustness by cell competition via mechano-chemical crosstalk
This research aims to understand the mechanisms by which tissues autonomously eliminate abnormal cells which occur naturally via cell competition and maintain robust tissue patterning. Focusing on the mechanical interaction between abnormal cells and neighboring fit cells, we will elucidate a new physicochemical basis for intercellular communication, in which abnormalities in various chemical signals are converted into the change of intercellular mechanical force and sensed by neighboring fit cells.
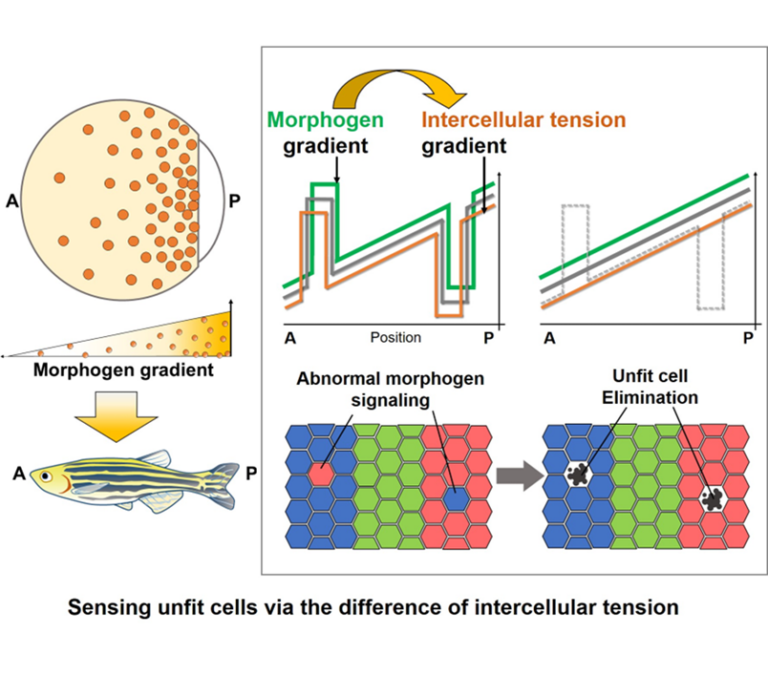
A03-2
Elucidating the mechanisms underlying cardiac lumen morphogenesis by blood flow forces
Cardiac lumen constantly undergoes physical stresses, such as blood flow and myocardial contraction, from the heart morphogenesis. Our studies have identified that mechano-responsive signals happen in both spatial and temporal manner during the cardiac lumen formation. However, the mechanism by which the cells appropriately regulate mechanical signals in response to the continuous forces is still unclear. In this project, we aim to elucidate how the structured cardiac lumen is organized for functional blood circulation, focusing on the “forces generated from blood flow” and “biological signals” during the lumen formation, and how cells discriminate the characteristics of forces. Specifically, we develop a technique that directly manipulates blood flow forces in zebrafish embryos and evaluate the biological signal outputs in response to forces by the quantitative imaging approach.
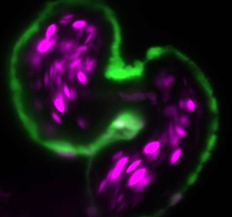
A03-3
Mehcano-chemical feedback mechanisms underlying zippering and neural tube closure in a simple chordate
My research seeks to address how self-organized collective cell movements emerge through the dynamic interplay of cell-cell signaling, force generation and tissue remodeling during embryonic development. Ascidians are marine invertebrate chordates, that are the closest relatives of vertebrates. They provide the opportunity to both manipulate and observe the dynamics of signaling, cell contractility and adhesion from subcellular to tissue scales, in the context of simple and highly stereotyped morphogenetic events with very few large cells in optically clear embryos. Our recent results suggest that a separation of cell-cell contact induce activation of actomyosin contractility for zippering and neural tube closure in ascidian embryos. In this team of the Transformative (A) research area, I will elucidate how the separation of cell-cell contacts is translated into activation of actomyosin contractility during ascidian neural tube closure.
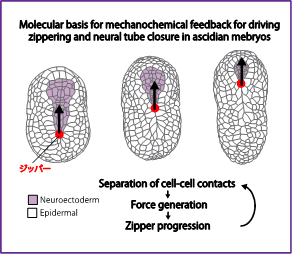
A03-4
Elucidation of Self-Transformation and Forces that Control Body Axis Rotation of Tailbud Embryo
Since multiple organs are involved in the shaping of the entire embryo during the organogenesis phase, the understanding of mechano-developmental mechanism is still unknown. The ascidian provides an interesting model to investigate how interactions among multiple tissues or organs contribute to the evolutionary conserved embryo shape in chordates. This study aims to elucidate the mechanism of ascidian tailbud embryo morphogenesis at trans-scale level, with a focus on body axis rotation observed in chordate embryos during the organogenesis period.
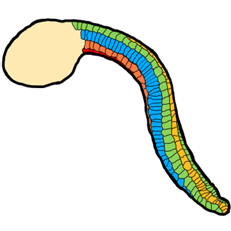
A03-5
Long-range mechanical signals that regulate organogenesis
Peristaltic waves, contractile waves of smooth muscles, propagate along gut tube over long distances, which potentially act as long-range mechanical signals. Using optogenetics and live imaging in chicken embryonic gut I investigate the mechanism in which the peristaltic waves regulate gut organogenesis such as gut tube elongation and villi formation. Through this project, I aim to propose the fundamental principle for accounting macro-scale morphogenesis.
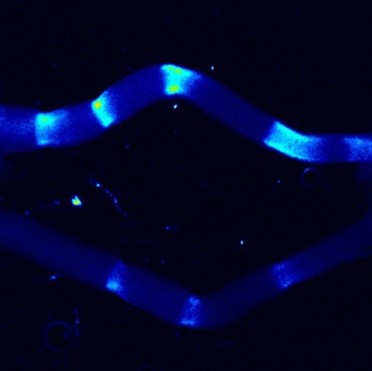
A03-6
Active gel physics for self-organized patterns and mechanochemical cross-talk in confined cytoskeletal systems
The contractile forces exerted by the actomyosin cytoskeleton can influence intracellular symmetry and non-equilibrium dynamics, ranging from single cells to biological tissues. However, bridging the microscopic forces to the macroscopic cellular ordering remains a significant challenge in the field of active matter physics. In this study, we investigate the physics of active actomyosin gels to elucidate the mechanisms underlying force-induced structural organization. Furthermore, we aim to extend this approach to a theoretical model of cell populations, shedding light on the principles of force-mediated collective ordering.
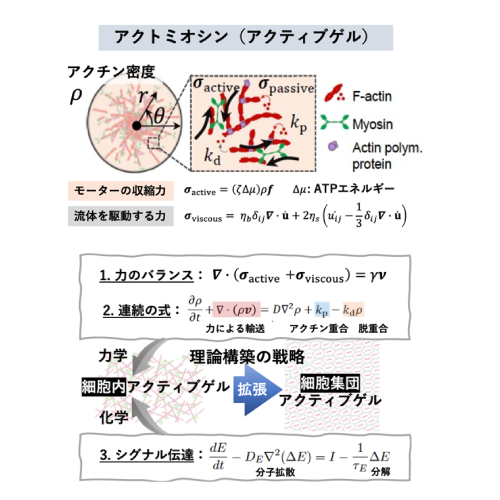
A03-7
Molecular coordination mechanisms between mechanical forces and chemical reaction during mammalian neural tube closure
Our group has studied mechanisms of mouse neural tube closure; in particular the role of neural folds which are located between neural ectoderm and surface ectoderm. Before the closure, these cells in the neural folds have initially uncommitted characters in which neither neural nor surface ectoderm markers are expressed but stem cell markers are. Grainyhead-like (GRHL)3 protein expresses specifically in these uncommitted progenitors in the nucleus and directs epidermal differentiation by transactivating epidermis-specific genes such as E-cadherin under the control of canonical Wnt signaling. Upon epidermal differentiation, the GRHL3 protein is localized in the cytoplasm and activates non-canonical Wnt signaling. Thereby, the cellular architecture of the epidermis has highly enriched actomyosin networks and the cell cortex becomes much stiffer. This project aims to understand how GRHL3-positive neural-fold cells sense mechanical forces within neural and surface epithelial sheets and integrate them into two major cellular biochemical signaling pathways at the molecular level during mammalian neural tube closure.
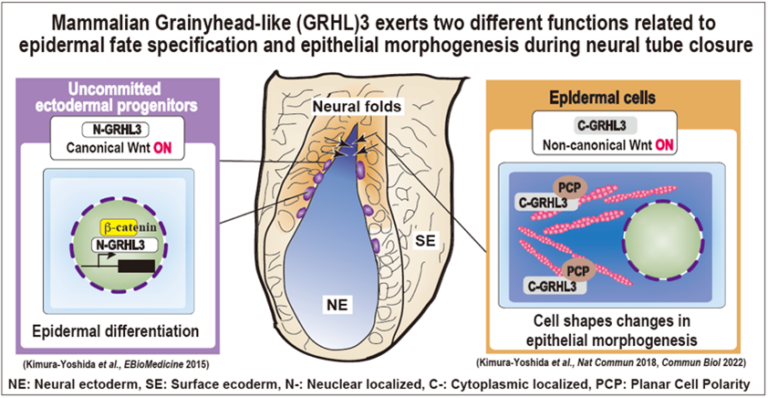
A03-8
Relationship between cellular mechanosensation and polarized cell/tissue dynamics during organ morphogenesis
In this study, we aim to clarify the connection between cellular mechanosensation and polarized cell/tissue dynamics, which plays a critical role in shaping organ-specific morphology. Specifically, we are focusing on the early developmental stages of the forebrain and heart to uncover shared morphogenetic mechanisms between organs.
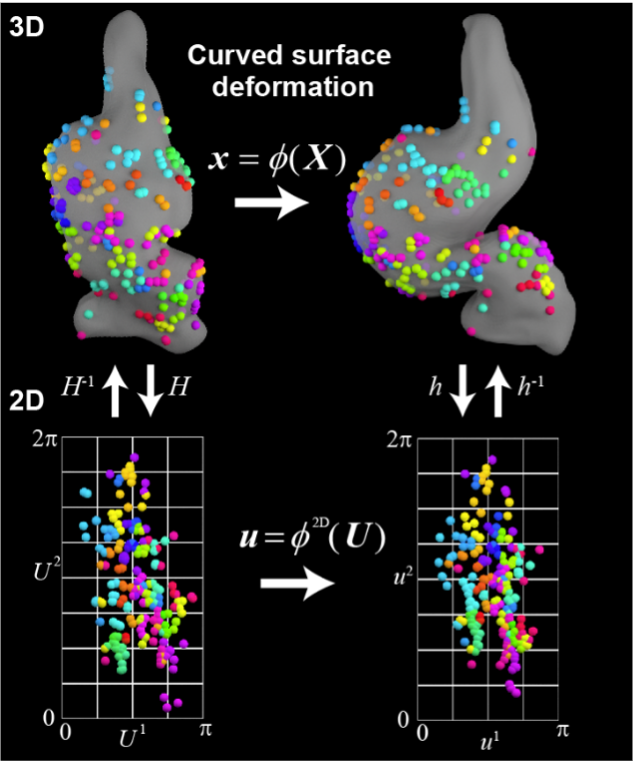
A03-9
Mechanical feedback system that allows for directional growth and posture control in plants
Morphogenesis inevitably involves instability because of the constant changes in shape and size. To cope with this constant spatiotemporal change and to ensure robust organogenesis, the proprioception mechanism is required for the recognition of the relative position of each body part and to provide a feedback to the overall shape. In plants, mechanical feedback has been proposed to coordinate the growth of individual cells and the entire organ. In this feedback, tension generated during morphogenesis serves as a signal and changes the orientation of microtubules, but the overall mechanism is still unknown. Here, we will elucidate the mechanism of microtubule alignment to the tensile stress, which is the key to mechanical feedback. My laboratory has previously found a NIMA-related kinase that has a unique property of changing its subcellular localization in response to tensile force. We will use the NIMA-related kinase as a clue for imaging the tension response and analyzing the mechanism of mechanical feedback. We will also search for novel factors that regulate the mechanical feedback.
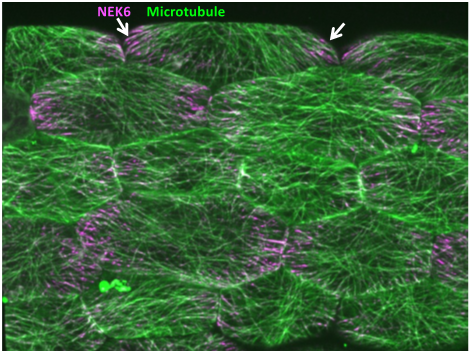
A03-10
Investigating the mechanical-chemical crosstalk that controls organismal reorganization in basal metazoans
This research aims to elucidate the nature of the mechanochemical crosstalk and self-organization mechanisms that enable organismal-level regeneration, or reorganization, in basal metazoans, using the jellyfish Cladonema. I will investigate the mechanisms of pattern formation during organismal reorganization at cellular and molecular levels and pursue the universal principles of self-organization that can be common to higher animals.
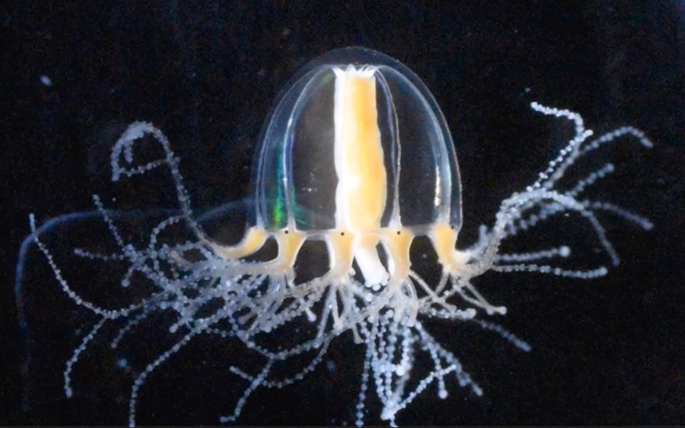
A03-11
The mechanical control of tissue regeneration by YAP mechano-homeostasis
When a part of the organ is lost, how do cells in an organ sense the whole organ size, proliferate and differentiate to restore the original organ size? How do cells stop doing so when the original organ size is restored by regeneration?
Our analysis of the “hirame” medaka fish mutant whose body and organs become flat due to its inability to withstand external forces uncovered the mechanism by which YAP generates an 3D organ that withstand external forces. YAP mediated cell tension control coordinates 3D tissue formation by cell stacking and subsequent 3D tissue alignment for generating a complex organ and the 3D body shape. The latter is mediated by YAP through the bidirectional mechanical control between a cell and extracellular matrix (ECM), which maintains tissue homeostasis (therefore, we named it YAP-mechanohomeostasis: this is the mechanism “You push me, I push you back.”).
Since YAP is required for regeneration, we aim to uncover how YAP-mechano-homeostasis contributes to regeneration by analyzing dynamics of YAP activation, ECM mechanics and mechanical response during regeneration in this study.
A03-12
Roles for intraventricular pressure in brain development
We explore roles for internal pressure of the brain ventricles mediated by cerebrospinal fluid (CSF) in brain development. We particularly focus on the transition from proliferative to neurogenic mode of the neural progenitor cells and on the expansion of the neural progenitor pool. Our research includes development of a method to measure the intraventricular pressure of different vertebrate species. We hope that notions obtained will also provide insights into brain evolution, disease, and organoid technologies.
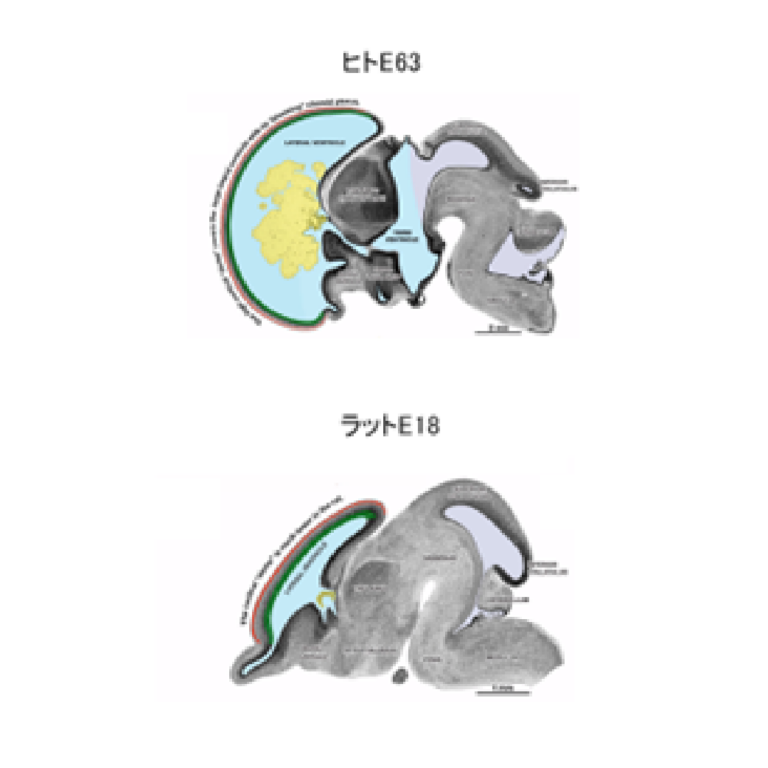
A03-13
Elucidation of the elongation mechanism of the entire limb bud by analysis of force-tissue interaction
Our research uses the limb bud of the chick embryo as a model system and analyzes the force interaction between the epithelium and mesenchyme of the limb bud, which are different tissues. We aim to elucidate the “mechanism by which the organs of the entire limb bud extend autonomously along the distance axis”. In our previous research, we found that the mechanical stress is five times higher along the anteroposterior axis, which is perpendicular to the elongation direction, and that there is stress anisotropy inside the epithelial tissue of the limb bud. Therefore, in this research, we will clarify the above from the following two perspectives, based on macro and micro level. (1) Macroscopic view: Using tissue culture, spatio-temporal quantitative analysis of the stress inside the epithelial tissue of the limb bud and measurement of the physical properties (Young’s modulus) of the epithelial tissue revealed compressive force to the entire mesenchymal cells inside. (2) Microscopic perspective: In order to understand the relationship between force and cell response and the mechanism that generates force at the cellular level, the cellular dynamics of epithelial tissue that produces stress anisotropy, the localization of molecules responsible for force. We will analyze changes in the order of cells in the mesenchyme (anisotropy of cell populations) at the micro level. In the field of developmental biology, research on the elongation mechanism of limb buds has been conducted mainly based on the expression of secretory factors, the tissue interaction between the epithelium and mesenchyme, and the analysis of the genes involved in it. Through this research, the concept of inter-tissue interaction has been reconsidered and redefined as “inter-tissue interaction including force exchange between the epithelium and mesenchyme”, and by redefining it, the entire macroscopic limb bud elongation mechanism will be clarified.
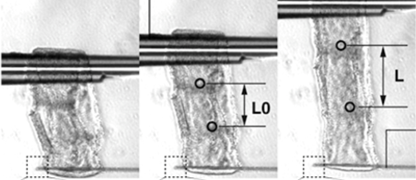